The vacuolar anti- Pseudomonal activity of neutrophil primary granule peptidyl-arginine deiminase enzymes - PubMed
- ️Mon Jan 01 2024
The vacuolar anti- Pseudomonal activity of neutrophil primary granule peptidyl-arginine deiminase enzymes
Rory Baird et al. Front Immunol. 2024.
Abstract
The role of neutrophils in host defense involves several cell processes including phagocytosis, degranulation of antimicrobial proteins, and the release of neutrophil extracellular traps (NETs). In turn, dysregulated cell activity is associated with the pathogenesis of airway and rheumatic diseases, in which neutrophil-derived enzymes including peptidyl-arginine deiminases (PADs) play a role. Known physiological functions of PADs in neutrophils are limited to the activity of PAD isotype 4 in histone citrullination in NET formation. The aim of this study was to extend our knowledge on the role of PADs in neutrophils and, specifically, bacterial killing within the confines of the phagocytic vacuole. Human neutrophils were fractionated by sucrose gradient ultracentrifuge and PADs localized in subcellular compartments by Western blot analysis. Direct interaction of PADs with Pseudomonas aeruginosa (P. aeruginosa) was assessed by flow cytometry and Western blot overlay. The participation of neutrophil PAD2 and PAD4 in killing of P. aeruginosa was assessed by inclusion of PAD-specific inhibitors. In vitro, bactericidal activity of recombinant human PAD2 or PAD4 enzymes against P. aeruginosa was determined by enumeration of colony-forming units (CFU). Together with neutrophil elastase (NE), PAD2 and PAD4 were localized to primary granules and, following activation with particulate stimuli, were degranulated in to the phagocytic vacuole. In vitro, PAD2 and PAD4 bound P. aeruginosa (p = 0.04) and significantly reduced bacterial survival to 49.1 ± 17.0 (p < 0.0001) and 48.5 ± 13.9% (p < 0.0001), respectively. Higher antibacterial activity was observed at neutral pH levels with the maximum toxicity at pH 6.5 and pH 7.5, comparable to the effects of neutrophil bactericidal permeability increasing protein. In phagosomal killing assays, inclusion of the PAD2 inhibitor, AFM-30a, or PAD4 inhibitor, GSK484, significantly increased survival of P. aeruginosa (AFM-30a, p = 0.05; and GSK484, p = 0.0079). Results indicate that PAD2 and PAD4 possess antimicrobial activity and are directly involved in the neutrophil antimicrobial processes. This study supports further research into the development of PAD-based antimicrobials.
Keywords: antimicrobial activity; bactericidal permeability increasing protein; neutrophils; peptidyl-arginine deiminases; phagocytic vacuole; primary granules.
Copyright © 2024 Baird, Yusuf, Forde, Pohl, Kavanagh, Fitzpatrick, Gogoi and Reeves.
Conflict of interest statement
The authors declare that the research was conducted in the absence of any commercial or financial relationships that could be construed as a potential conflict of interest.
Figures
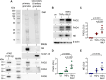
PAD2 and PAD4 are present in primary granules and degranulated extracellularly in response to soluble stimuli. (A) Neutrophil granules were isolated by sucrose gradient ultracentrifugation of neutrophil lysates (5 × 107). Coomassie Blue stain of polyacrylamide gel loaded with neutrophil primary granules and secondary and tertiary granules. Western blot detection of PADs in respective fractions, including PAD6 (negative control), primary granule marker neutrophil elastase (NE), and secondary granule marker hCAP-18. Recombinant human proteins of PAD isotypes loaded (1.25 ng) to the left of the molecular weight marker (MWM) as positive controls. Representative images from n = 6 biological repeats. (B–E) Neutrophils (1.5 × 107) were left unstimulated (un) or stimulated for 10 min with TNFα or TNFα and fMLP. Extracellular supernatants were assessed by Western blot for PAD2, PAD4, and the primary granule marker, NE. Neutrophil pellets were probed for β-actin (loading control). (C–E) Abundance of extracellular PADs was quantified by densitometric analysis of immunobands. Data are expressed as relative densitometry units (DU), with representative Western blots presented (N = 6 biological repeats, one-way ANOVA, followed by Tukey’s post-hoc multiple comparison test).
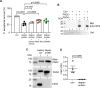
PAD2 and PAD4 released extracellularly impact bacterial killing independent of NET formation. (A) Survival of P. aeruginosa after 10 min exposure to degranulated proteins obtained from TNFα (10 ng/mL) and fMLP (1 µM) stimulated neutrophils (1.5 × 107, 10 min of stimulation). Extracellular supernatants were treated with DMSO (vehicle control), AMF-30a (PAD2-inhibitor), or GSK484 (PAD4-inhibitor) (50 µM). Data were analyzed by one-way ANOVA followed by multiple comparison Tukey’s post-hoc test and presented as mean ± SEM, n = 5 biological replicates. (B) Representative Western blot showing citrullination of Histone H3 (CitH3) by PAD2 and PAD4 in the presence of Ca2+ (1 mM). Lower panel is a Coomassie Blue–stained gel of reactions, demonstrating equal H3 protein loading. (C) Neutrophils (1.5 × 107) were stimulated for 10 min with TNFα and fMLP. Extracellular supernatants of activated cells were probed by Western blot for PAD2, PAD4, and the NET marker CitH3 (right hand lanes). CitH3 was not detected, confirming the absence of NETs in supernatants of activated neutrophils. Loading controls in the left hand lane include rPAD2, rPAD4, and CitH3. (D) By densitometric analysis, CitH3 was not detected extracellularly. Data are expressed as relative densitometry units (DU) (N = 4 biological repeats).
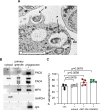
PAD2 and PAD4 are degranulated into the bacterial phagosome and participate in bacterial killing. (A) Representative micrograph showing neutrophil forming pseudopodia at the time of engulfment and bacteria inside phagocytic vacuoles following 3 min of incubation [Labels indicate bacteria (B), phagosomes (P), and plasma membrane (PM); scale bar, 2 μm]. (B) Western blot analysis of neutrophil cytosol, primary granules and phagosome fractions for PADs, including primary granule and phagosome marker MPO, and cytosolic marker GAPDH. Representative images of n = 3 biological replicates. (C) P. aeruginosa survival following 30 min of neutrophil (5 × 107/mL) phagosomal killing (MOI = 4; 1 neutrophil to 4 bacteria) (con). Neutrophils were pre-treated with PAD inhibitors AMF-30a (50 µM) or GSK484 (50 µM) for 15 min prior to killing assays. DMSO [0.5% (v/v)] was used as the solvent control. Data were analyzed by a one-way ANOVA followed by multiple comparison Tukey’s post-hoc test and presented as mean ± SEM, n = 5 biological replicates.
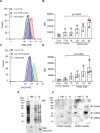
PAD2 and PAD4 directly bind P. aeruginosa. Flow cytometry analysis of PAD2 (A, B) and PAD4 (C, D) (10–80 nM) membrane interaction with P. aeruginosa (5 × 107 CFU) after 30 min of incubation at 37°C. rPAD2 and rPAD4 binding was evaluated using anti-PAD2 and anti-PAD4 rabbit polyclonal antibodies, respectfully, followed by use of anti-rabbit PE-conjugated antibody. Data were analyzed by one-way ANOVA followed by multiple comparison Tukey’s post-hoc test and presented as mean ± SEM, n = 3 biological replicates. (E) Subcellular fractions of P. aeruginosa [bacterial whole-cell lysate (L), cytosol (C), and membrane proteins (M)] were electrophoresed on a polyacrylamide gel and stained with Coomassie Blue (top panel). A Western blot for P. aeruginosa LPS confirmed purity of membrane proteins (lower panel). (F) The subcellular distribution of the bacterial adhesion to which PADs bound was determined after transfer to PVDF membrane and incubation with rPAD2 or rPAD4. Arrows indicate protein bands of ∼60, 42, and 26 kilodaltons in the outer membrane fractions corresponding to molecules with which rPAD2 and rPAD4 interacted.
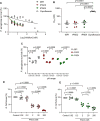
Recombinant human PAD2 and PAD demonstrate dose-dependent killing of P. aeruginosa and E coli. (A) Survival of P. aeruginosa (5 × 105 CFU/mL) 30 min after incubation with rPAD2, rPAD4, rBPI (2.5–40 nM), or ciprofloxacin (2.5–1500 nM) in PBS (pH 7.4). Survival (%) calculated relative to untreated P. aeruginosa. Data expressed as a non-linear regression and presented as mean ± SEM, n = 5 biological replicates repeats. (B) Compounds were tested for activity against P. aeruginosa and MIC50 values calculated. (C) Survival of P. aeruginosa (5 × 105 CFU/mL) 30 min after incubation with rPAD2, rPAD4, or rBPI (20 nM) in PBS (pH 5.5, 6.5, 7.5, or 8.5). Survival (%) calculated relative to P. aeruginosa CFU/mL in 0 nM enzyme at respective pH levels. (D, E) Survival of E Coli (5 × 105 CFU/mL) 60 min after incubation with rPAD2 (D) or rPAD4 (E) in PBS (pH 7.4) (n = 5 biological replicates). Data were analyzed by one-way or two-way ANOVA, followed by multiple comparison Tukey’s post-hoc test and presented as mean ± SEM.
Similar articles
-
Domiciano TP, Lee Y, Carvalho TT, Wakita D, Martinon D, Jena PK, Fert-Bober J, Borges V, Crother TR, Chen S, Moreira D, Van Eyk JE, Noval Rivas M, Arditi M, Shimada K. Domiciano TP, et al. Clin Exp Immunol. 2024 Nov 12;218(3):314-328. doi: 10.1093/cei/uxae080. Clin Exp Immunol. 2024. PMID: 39250707
-
Martín Monreal MT, Rebak AS, Massarenti L, Mondal S, Šenolt L, Ødum N, Nielsen ML, Thompson PR, Nielsen CH, Damgaard D. Martín Monreal MT, et al. Front Immunol. 2021 Oct 19;12:716250. doi: 10.3389/fimmu.2021.716250. eCollection 2021. Front Immunol. 2021. PMID: 34737738 Free PMC article.
-
Murphy MP, Hunt D, Herron M, McDonnell J, Alshuhoumi R, McGarvey LP, Fabré A, O'Brien H, McCarthy C, Martin SL, McElvaney NG, Reeves EP. Murphy MP, et al. J Immunol. 2024 Jul 1;213(1):75-85. doi: 10.4049/jimmunol.2300658. J Immunol. 2024. PMID: 38758115 Free PMC article.
-
Ho JW, Quan C, Gauger MA, Alam HB, Li Y. Ho JW, et al. Shock. 2023 Feb 1;59(2):247-255. doi: 10.1097/SHK.0000000000002052. Epub 2023 Jan 5. Shock. 2023. PMID: 36597759 Free PMC article. Review.
-
The roles of PAD2- and PAD4-mediated protein citrullination catalysis in cancers.
Wang Y, Chen R, Gan Y, Ying S. Wang Y, et al. Int J Cancer. 2021 Jan 15;148(2):267-276. doi: 10.1002/ijc.33205. Epub 2020 Aug 8. Int J Cancer. 2021. PMID: 33459350 Review.
References
MeSH terms
Substances
Grants and funding
The author(s) declare financial support was received for the research, authorship, and/or publication of this article. The authors declare that this study received funding from the Irish Research Council (Grant # GOIPG/2023/3100) and research Fellowship funding from Pfizer Healthcare Ireland (2021). The funders were not involved in the study design, collection, analysis, interpretation of data, the writing of this article or the decision to submit it for publication.
LinkOut - more resources
Full Text Sources
Miscellaneous