Phase locking to high frequencies in the auditory nerve and cochlear nucleus magnocellularis of the barn owl, Tyto alba - PubMed
- ️Wed Jan 01 1997
Phase locking to high frequencies in the auditory nerve and cochlear nucleus magnocellularis of the barn owl, Tyto alba
C Köppl. J Neurosci. 1997.
Abstract
The auditory system of the barn owl is an important model for temporal processing on a very fast time scale and for the neural mechanisms and circuitry underlying sound localization. Phase locking has been shown to be the behaviorally relevant temporal code. This study examined the quality and intensity dependence of phase locking in single auditory nerve fibers of the barn owl to define the input to the known brainstem circuit for temporal processing. For direct comparison in the same individuals, recordings were also obtained from the relevant next higher center, the nucleus magnocellularis (NM). Phase locking was regularly seen at sound pressure levels (SPL) below those eliciting an increase in spike rate, thus providing an additional cue for signal detection. The quality of phase locking, expressed as vector strength, decreased with increasing frequency. Auditory nerve fibers showed an unusual step-like decline with a prominent plateau in the mid-frequency range (1.5-3 kHz), indicating that some specialization enables the owl to halt the deterioration and extend phase locking to frequencies up to 10 kHz, above the range commonly observed in other species. Phase locking in the NM was consistently inferior to that of auditory-nerve fibers at frequencies above 1 kHz, suggesting that the synapse plays a limiting role in temporal precision. The response delays, or group delays, derived from the phase-versus-frequency functions of auditory nerve fibers were not consistent with the unusual spatial frequency representation in the owl cochlea. This questions the common assumption that group delays reflect cochlear wave travel times.
Figures
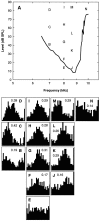
Example of phase locking in an auditory nerve fiber at frequencies near the upper end of the frequency range over which the owl is sensitive. A, Rate threshold tuning curve of the fiber; the CF was 8.7 kHz. The positions of the lettersB–N indicate the frequencies and levels of stimuli whose corresponding phase histograms are shown in panelsB-N. Phase histograms showing the number of spikes (ordinates, all scaled identically) that occurred in different time bins relative to the zero-crossing of the sinusoidal stimulus; each histogram covers one stimulus period along theabscissa. All stimuli (except the subthreshold one inE) produced statistically significant phase locking; the vector strength is given in each panel.
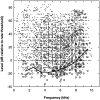
Frequency and relative level above rate threshold of all stimuli tested for phase locking in all auditory nerve fibers. Stimuli that elicited significant phase locking are shown asdots (n = 3115), those that failed to elicit phase locking as open circles(n = 254). The thin dashed lineindicates the rate threshold level. As a measure for phase-locking thresholds as a function of frequency, the median relative levels of all stimuli (in 1 kHz-bins) that did not result in phase locking are connected by the thick solid line. Note that the phase-locking threshold lies 15–20 dB below the rate threshold up to 6 kHz, but approaches the rate threshold and even lies above it toward the upper end of the frequency range of the owl.
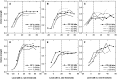
A–F, Examples of the growth of vector strength with rising stimulus level in neurons of different CF.A–C, Data from auditory nerve fibers;D–F, data from NM units. Each panel shows several curves from a single unit at the different stimulus frequencies indicated. Only stimulus levels producing significant phase locking are included. A vertical dashed line in each panel indicates the level above which saturation of vector strength was generally assumed and values were included in Figure 4.
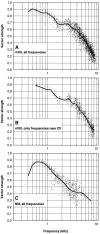
Vector strength as a function of stimulus frequency. Only values for stimuli whose level was at least 20 dB above the rate threshold, i.e., where vector strength was saturated, are shown. The solid lines connect the median values calculated for quarter octave bins. A, All data from auditory nerve fibers (n = 1947). B, Data from auditory nerve fibers but restricted to stimuli close to CF (CF ± 0.1 octaves for CFs up to 5 kHz, CF ± 0.05 octaves for CFs above 5 kHz; n = 661). C, All data from NM units (n = 572).
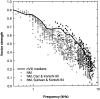
A comparison of saturated vector strength as a function of frequency, as determined by different investigators in the auditory nerve and NM of the barn owl. The thick line represents median values for the auditory nerve as shown in Figure4A. Open circles repeat the NM data shown in Figure 4C. Open trianglesare NM data shown in Figure 5D of Carr and Konishi (1990). Filled squares are the NM data shown in Figure 2 of Sullivan and Konishi (1984). Note that the majority of NM data lies below the median curve for the auditory nerve data.
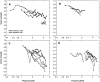
Saturated vector strength as a function of stimulus frequency for individual neurons. Each of the panelsA–D shows several examples of neurons from an individual owl; curves from auditory nerve fibers are drawn withfilled symbols, those from NM units with open symbols. Note that vector strengths of NM units are regularly lower in the mid-frequency range and that several auditory nerve functions show a plateau of almost constant vector strength values between 2 and 3 kHz.
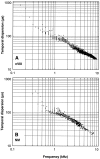
Temporal dispersion as a function of stimulus frequency. Temporal dispersion was derived from the vector strengths shown in Figure 4, A and C, respectively (for details see Results) A, Data from auditory nerve fibers. B, Data from NM units.
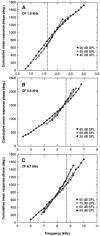
A–C, Three examples of the change in phase-versus-frequency functions with changes in sound pressure level. A, Curves from a low frequency NM unit.B, C, Curves from a mid- and high-frequency auditory nerve fiber, respectively. The cumulative mean response phase is plotted as a function of stimulus frequency. Thevertical dashed line in each panel indicates the CF of the neuron. Note the tilting of the phase-versus-frequency functions around a frequency with the most stable mean response phase, which changes from lying above the CF in the low CF neuron (A) to below the CF in the high CF neuron (C).
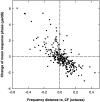
The rate of change of the mean response phase with level, as a function of the distance of the stimulus frequency from the respective CF. Only data from auditory nerve fibers are shown (n = 265).
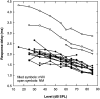
The response delay (derived from the slope of the phase-versus-frequency function, see Materials and Methods) as a function of stimulus sound pressure level. The linesconnect data from individual neurons; those from auditory nerve fibers are drawn with filled symbols, those from NM units withopen symbols. Note that the response delay decreases approximately linearly with rising sound pressure level.
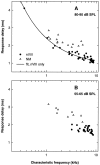
The response delay as a function of CF. Data from auditory nerve fibers are drawn as filled circles, those from NM units as open triangles. A, Delays derived from phase-versus-frequency functions obtained at 80–90 dB SPL stimulus level. The solid line shows the power fit to the auditory nerve data only (delay = 205CF (in Hz)−0.706 + 0.833;n = 40). B, Delays derived from data obtained with a lower stimulus level of 55–65 dB SPL.
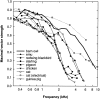
A comparison of maximal vector strength as a function of frequency in the auditory nerve of several avian and mammalian species. For the bird species, median values in mostly quarter octave bins are shown, taken or calculated from the following sources: barn owl, data from Figure 4A; emu, Figure 9 in Manley et al. (1997); redwing blackbird, Figure11-9B in Sachs et al. (1980); starling, Figure 4 in Gleich and Narins (1988); pigeon, graphic estimate of median values from Figure 2 in Hill et al. (1989); chicken, Figure 16 from Salvi et al. (1992). For the cat and guinea pig, average values in 0.1 decade bins as determined by Weiss and Rose (1988a, their Fig. 3) from the original data (Johnson, 1980; Palmer and Russell, 1986) are shown. The two different data sets for electrical stimulation in the cat (Hartmann and Klinke, 1987; Dynes and Delgutte, 1992) are mean values taken from Figure 10 in Dynes and Delgutte (1992).
Similar articles
-
Köppl C. Köppl C. J Comp Neurol. 1994 Jan 15;339(3):438-46. doi: 10.1002/cne.903390310. J Comp Neurol. 1994. PMID: 8132870
-
Encoding of amplitude modulation in the cochlear nucleus of the cat.
Rhode WS, Greenberg S. Rhode WS, et al. J Neurophysiol. 1994 May;71(5):1797-825. doi: 10.1152/jn.1994.71.5.1797. J Neurophysiol. 1994. PMID: 8064349
-
Spike timing in auditory-nerve fibers during spontaneous activity and phase locking.
Heil P, Peterson AJ. Heil P, et al. Synapse. 2017 Jan;71(1):5-36. doi: 10.1002/syn.21925. Epub 2016 Aug 17. Synapse. 2017. PMID: 27466786 Review.
Cited by
-
The quantal component of synaptic transmission from sensory hair cells to the vestibular calyx.
Highstein SM, Mann MA, Holstein GR, Rabbitt RD. Highstein SM, et al. J Neurophysiol. 2015 Jun 1;113(10):3827-35. doi: 10.1152/jn.00055.2015. Epub 2015 Apr 15. J Neurophysiol. 2015. PMID: 25878150 Free PMC article.
-
The representation of sound localization cues in the barn owl's inferior colliculus.
Singheiser M, Gutfreund Y, Wagner H. Singheiser M, et al. Front Neural Circuits. 2012 Jul 11;6:45. doi: 10.3389/fncir.2012.00045. eCollection 2012. Front Neural Circuits. 2012. PMID: 22798945 Free PMC article.
-
Noise reduction of coincidence detector output by the inferior colliculus of the barn owl.
Christianson GB, Peña JL. Christianson GB, et al. J Neurosci. 2006 May 31;26(22):5948-54. doi: 10.1523/JNEUROSCI.0220-06.2006. J Neurosci. 2006. PMID: 16738236 Free PMC article.
-
Computational diversity in the cochlear nucleus angularis of the barn owl.
Köppl C, Carr CE. Köppl C, et al. J Neurophysiol. 2003 Apr;89(4):2313-29. doi: 10.1152/jn.00635.2002. Epub 2002 Dec 27. J Neurophysiol. 2003. PMID: 12612008 Free PMC article.
-
Prolonged maturation of cochlear function in the barn owl after hatching.
Köppl C, Nickel R. Köppl C, et al. J Comp Physiol A Neuroethol Sens Neural Behav Physiol. 2007 Jun;193(6):613-24. doi: 10.1007/s00359-007-0216-z. Epub 2007 Feb 24. J Comp Physiol A Neuroethol Sens Neural Behav Physiol. 2007. PMID: 17323066
References
-
- Anderson DJ, Rose JE, Hind JE, Brugge JF. Temporal position of discharges in single auditory nerve fibres within the cycle of a sine-wave stimulus: frequency and intensity effects. J Acoust Soc Am. 1971;49:1131–1139. - PubMed
-
- Cant NB. The cochlear nucleus: neuronal types and their synaptic organization. In: Webster DB, Popper AN, Fay RR, editors. The mammalian auditory pathway: neuroanatomy. Springer; New York: 1992. pp. 66–116.
-
- Carr CE. Evolution of the central auditory system in reptiles and birds. In: Webster DB, Fay RR, Popper AN, editors. The evolutionary biology of hearing, Ed 1. Springer; New York: 1992. pp. 511–543.
-
- Carr CE. Delay line models of sound localization in the barn owl. Am Zool. 1993a;33:79–85.
-
- Carr CE. Processing of temporal information in the brain. Annu Rev Neurosci. 1993b;16:223–243. - PubMed
Publication types
MeSH terms
LinkOut - more resources
Full Text Sources
Other Literature Sources
Miscellaneous