Evolution of genome size and complexity in Pinus - PubMed
- ️Invalid Date
Evolution of genome size and complexity in Pinus
Alison M Morse et al. PLoS One. 2009.
Abstract
Background: Genome evolution in the gymnosperm lineage of seed plants has given rise to many of the most complex and largest plant genomes, however the elements involved are poorly understood.
Methodology/principal findings: Gymny is a previously undescribed retrotransposon family in Pinus that is related to Athila elements in Arabidopsis. Gymny elements are dispersed throughout the modern Pinus genome and occupy a physical space at least the size of the Arabidopsis thaliana genome. In contrast to previously described retroelements in Pinus, the Gymny family was amplified or introduced after the divergence of pine and spruce (Picea). If retrotransposon expansions are responsible for genome size differences within the Pinaceae, as they are in angiosperms, then they have yet to be identified. In contrast, molecular divergence of Gymny retrotransposons together with other families of retrotransposons can account for the large genome complexity of pines along with protein-coding genic DNA, as revealed by massively parallel DNA sequence analysis of Cot fractionated genomic DNA.
Conclusions/significance: Most of the enormous genome complexity of pines can be explained by divergence of retrotransposons, however the elements responsible for genome size variation are yet to be identified. Genomic resources for Pinus including those reported here should assist in further defining whether and how the roles of retrotransposons differ in the evolution of angiosperm and gymnosperm genomes.
Conflict of interest statement
Competing Interests: The authors have declared that no competing interests exist.
Figures
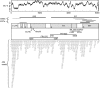
Percent G+C is shown above the Gymny schematic. Numbered ORFs are in gray with vertical lines indicating stop codons, putative 5′LTR as hatched box, PR protease, RT reverse transcriptase, INT integrase. ESTs, GSSs, and 454 reads are indicated as are the B8_650 marker, and the Southern probes Fr1075 and Fr1035.
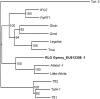
Relatedness tree generated from alignment of RT sequences in Figure S1 and the RT domain from the Arabidopsis Ta1-3 Copia-like retrotransposon (Accession number X13291; [38]). Bootstrap percent values based on 10,000 replications.
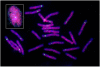
RLG_Gymny_EU912388-1 probes Fr1035 and Fr1075 were detected with Cy3 streptavidin (red) and 18S–28S rDNA was detected with FITC (green). Inset shows FISH to interphase nucleus.
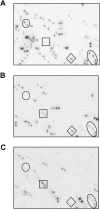
Clones are spotted in duplicate so positive signal is a closely situated pair of spots. BACs within circles, squares, diamonds and ovals show differential hybridization among probes. While most symbols highlight a single BAC (i.e. a pair of spots), the oval highlights two BACs that hybridized to all three overgo probes.
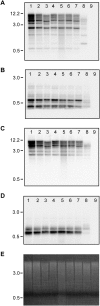
The location of probes Fr1035 and Fr1075 in RLG_Gymny_EU912388-1 are indicated in Figure 1. (A, B) filters hybridized with Fr1075 probe; (C, D) filters hybridized with Fr1035 probe; (A, C) digested with HindIII; (B, D) digested with HaeIII; (E) representative HindIII digested DNA stained with ethidium bromide. Lanes (1) Pinus glabra, (2) P. taeda, (3) P. elliottii, (4) P. radiata, (5) P. echinata, (6) P. palustris, (7) P. virginiana, (8) P. pinea, (9) P. strobus.
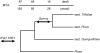
The tree shown is derived from the analyses performed by Willyard et al. where the dates for the nodes were selected to show the maximum possible range of values using either wood or leaf fossil calibrations, and either nuclear DNA or chloroplast DNA markers.
Similar articles
-
Pavy N, Pelgas B, Laroche J, Rigault P, Isabel N, Bousquet J. Pavy N, et al. BMC Biol. 2012 Oct 26;10:84. doi: 10.1186/1741-7007-10-84. BMC Biol. 2012. PMID: 23102090 Free PMC article.
-
Kirst M, Johnson AF, Baucom C, Ulrich E, Hubbard K, Staggs R, Paule C, Retzel E, Whetten R, Sederoff R. Kirst M, et al. Proc Natl Acad Sci U S A. 2003 Jun 10;100(12):7383-8. doi: 10.1073/pnas.1132171100. Epub 2003 May 27. Proc Natl Acad Sci U S A. 2003. PMID: 12771380 Free PMC article.
-
Wright DA, Voytas DF. Wright DA, et al. Genetics. 1998 Jun;149(2):703-15. doi: 10.1093/genetics/149.2.703. Genetics. 1998. PMID: 9611185 Free PMC article.
-
Insights into conifer giga-genomes.
De La Torre AR, Birol I, Bousquet J, Ingvarsson PK, Jansson S, Jones SJ, Keeling CI, MacKay J, Nilsson O, Ritland K, Street N, Yanchuk A, Zerbe P, Bohlmann J. De La Torre AR, et al. Plant Physiol. 2014 Dec;166(4):1724-32. doi: 10.1104/pp.114.248708. Epub 2014 Oct 27. Plant Physiol. 2014. PMID: 25349325 Free PMC article. Review.
-
The epigenetic control of the Athila family of retrotransposons in Arabidopsis.
Slotkin RK. Slotkin RK. Epigenetics. 2010 Aug 16;5(6):483-90. doi: 10.4161/epi.5.6.12119. Epub 2010 Aug 16. Epigenetics. 2010. PMID: 20484989 Review.
Cited by
-
Comparative transcriptomics of a complex of four European pine species.
Wachowiak W, Trivedi U, Perry A, Cavers S. Wachowiak W, et al. BMC Genomics. 2015 Mar 25;16(1):234. doi: 10.1186/s12864-015-1401-z. BMC Genomics. 2015. PMID: 25887584 Free PMC article.
-
Raherison E, Rigault P, Caron S, Poulin PL, Boyle B, Verta JP, Giguère I, Bomal C, Bohlmann J, MacKay J. Raherison E, et al. BMC Genomics. 2012 Aug 29;13:434. doi: 10.1186/1471-2164-13-434. BMC Genomics. 2012. PMID: 22931377 Free PMC article.
-
The first insight into the Taxus genome via fosmid library construction and end sequencing.
Hao D, Yang L, Xiao P. Hao D, et al. Mol Genet Genomics. 2011 Mar;285(3):197-205. doi: 10.1007/s00438-010-0598-4. Epub 2011 Jan 5. Mol Genet Genomics. 2011. PMID: 21207064
-
Comparative Transcriptomics Among Four White Pine Species.
Baker EAG, Wegrzyn JL, Sezen UU, Falk T, Maloney PE, Vogler DR, Delfino-Mix A, Jensen C, Mitton J, Wright J, Knaus B, Rai H, Cronn R, Gonzalez-Ibeas D, Vasquez-Gross HA, Famula RA, Liu JJ, Kueppers LM, Neale DB. Baker EAG, et al. G3 (Bethesda). 2018 May 4;8(5):1461-1474. doi: 10.1534/g3.118.200257. G3 (Bethesda). 2018. PMID: 29559535 Free PMC article.
-
Evolution of exon-intron structure and alternative splicing.
Koralewski TE, Krutovsky KV. Koralewski TE, et al. PLoS One. 2011 Mar 25;6(3):e18055. doi: 10.1371/journal.pone.0018055. PLoS One. 2011. PMID: 21464961 Free PMC article.
References
-
- Grotkopp E, Rejmanek M, Sanderson MJ, Rost TL. Evolution of genome size in pines (Pinus) and its life-history correlates: Supertree analyses. Evolution. 2004;58:1705–1729. - PubMed
-
- Price RA, Liston A, Strauss SH. Phylogeny and systematics of Pinus. In: Richardson DM, editor. Ecology and Biogeography of Pinus. Cambridge: Cambridge University Press; 1989. pp. 49–68.
-
- Gernandt DS, Lopez GG, Garcia SO, Liston A. Phylogeny and classification of Pinus. Taxon. 2005;54:29–42.
-
- Dvorak WS, Jordon AP, Hodge GP, Romero JL. Assessing evolutionary relationships of pines in the Oocarpae and Australes subsections using RAPD markers. New Forests. 2000;20:163–192.
-
- McKeand S, Mullin T, Byram T, White T. Deployment of genetically improved loblolly and slash pines in the south. Journal of Forestry. 2003;101:32–37.
Publication types
MeSH terms
Substances
LinkOut - more resources
Full Text Sources
Miscellaneous