Interplay of metal ions and urease - PubMed
Review
Interplay of metal ions and urease
Eric L Carter et al. Metallomics. 2009.
Abstract
Urease, the first enzyme to be crystallized, contains a dinuclear nickel metallocenter that catalyzes the decomposition of urea to produce ammonia, a reaction of great agricultural and medical importance. Several mechanisms of urease catalysis have been proposed on the basis of enzyme crystal structures, model complexes, and computational efforts, but the precise steps in catalysis and the requirement of nickel versus other metals remain unclear. Purified bacterial urease is partially activated via incubation with carbon dioxide plus nickel ions; however, in vitro activation also has been achieved with manganese and cobalt. In vivo activation of most ureases requires accessory proteins that function as nickel metallochaperones and GTP-dependent molecular chaperones or play other roles in the maturation process. In addition, some microorganisms control their levels of urease by metal ion-dependent regulatory mechanisms.
Figures
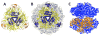
The structures of three well-characterized ureases. (A) K. aerogenes urease (PDB access code 1fwj) with UreA depicted in blue, UreB in orange, and UreC in yellow, together forming a (UreABC)3 structure. (B) H. pylori urease (1e9z) with UreA (corresponding to a fusion of the two small subunits in the K. aerogenes enzyme) depicted in blue and UreB (analogous to UreC in the K. aerogenes protein) shown in yellow for one (UreAB)3 unit, with three more (UreAB)3 units shown in gray included in the biologically relevant [(UreAB)3]4 structure. (C) Jack bean urease with one subunit (comparable to a fusion of all three K. aerogenes subunits) shown in gold in the otherwise blue hexameric protein (two trimers interacting in a face-to-face manner and shown after a 90 degree rotation compared to the other ureases). (Copied by permission of the International Union of Crystallography;
http://journals.iucr.org/).
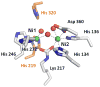
The urease active site. The active site of urease contains two nickel atoms (green) bridged by a carboxylated lysine and a hydroxyl group. Ni1 is also coordinated by two histidine residues and a solvent molecule, while Ni2 is coordinated by two histidines, an aspartic acid residue, and a water molecule. Waters are red, metal-binding side chains are shown with white carbon atoms, and two nearby histidine residues that function in catalysis are shown with orange carbon atoms.
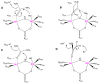
Proposals for the urease catalytic mechanism. (A) The hydroxyl group bound to Ni-2 attacks urea, whose carbonyl group is polarized by coordination to Ni1, forming a tetrahedral intermediate that releases ammonia with His 320 (K. aerogenes numbering) acting as a general acid. (B) The bridging hydroxyl group attacks urea, bound with its carbonyl group coordinated to Ni1 and an amine interacting with Ni2, and the hydroxyl proton transfers to the released ammonia. (C) A merged mechanism in which the bridging water attacks the substrate, but with His 320 acting as a general acid. (D) Elimination mechanism to form a cyanic acid (O=C=N-H) intermediate that subsequently becomes hydrated (not depicted) to form carbamate. In all mechanisms, the carbamate spontaneously decomposes.
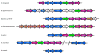
Organization of representative urease gene clusters. The urease gene cluster of K. aerogenes (ureDABCEFG) is compared to the gene organization found in selected other bacteria: R. leguminosarum, containing insertions of unidentified genes; Bacillus sp. TB-90, which repositions ureD and adds a likely nickel permease (ureH); A. pleuropneumoniae, which juxtaposes genes encoding a likely nickel transport (cbiKLMO) and urea permease (utp); Helicobacter species which fuse the two small structural subunits and add a proton-gated urea channel (ureI); H. mustelae containing a second, incomplete urease cluster; and B. subtilis that lacks urease accessory genes. Genes encoding urease subunits are shown in blue, the ureE gene encoding a metallochaperone is green, other urease accessory genes are purple, genes encoding proteins involved in nickel uptake are orange, those encoding proteins related to urea transport are red, and unknown genes are white. The sizes of the arrows do not accurately reflect the sizes of the genes.
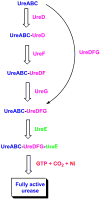
Postulated interactions among urease-related bacterial proteins. The urease apoprotein sequentially binds UreD, UreF, and UreG, where the in vitro activation properties exhibit differences in each apoprotein species. Alternatively, a preformed UreDFG complex may bind the apoprotein. Within the resulting complex, the UreDFG heterotrimer acts as GTP-dependent molecular chaperone to enhance exposure of the nascent active site. UreE interacts with the UreABC-UreDFG complex and delivers nickel ions, thus serving a metallochaperone role. Carbon dioxide is used to form the carboxy-lysine metal ligand, and GTP hydrolysis (occurring in UreG) drives the metallocenter assembly process to provide active urease, with release of all accessory proteins.
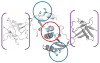
Structure of UreE. The structure of homodimeric K. aerogenes H144* UreE (PDB access code 1gmw), a truncated protein missing the C-terminal His-rich sequence) is depicted without metal ions. The central metal binding site (encircled in red) occurs at the subunit interface site and binds two nickel ions using, in part, His 96 residues from each subunit. Auxiliary metal binding sites (encircled in blue), involving the non-essential His 110 and His 112 residues, are presumed to donate nickel ions to the central site. When present, the C-terminal extension is also well positioned to provide nickel ions to the interfacial site. In addition to the metal-binding domain, a “peptide-binding domain” is present (in brackets) but is not essential for UreE function.
Similar articles
-
Yuen MH, Fong YH, Nim YS, Lau PH, Wong KB. Yuen MH, et al. Proc Natl Acad Sci U S A. 2017 Dec 19;114(51):E10890-E10898. doi: 10.1073/pnas.1712658114. Epub 2017 Dec 4. Proc Natl Acad Sci U S A. 2017. PMID: 29203664 Free PMC article.
-
Chemistry of Ni2+ in urease: sensing, trafficking, and catalysis.
Zambelli B, Musiani F, Benini S, Ciurli S. Zambelli B, et al. Acc Chem Res. 2011 Jul 19;44(7):520-30. doi: 10.1021/ar200041k. Epub 2011 May 4. Acc Chem Res. 2011. PMID: 21542631
-
Yang X, Li H, Lai TP, Sun H. Yang X, et al. J Biol Chem. 2015 May 15;290(20):12474-85. doi: 10.1074/jbc.M114.632364. Epub 2015 Mar 9. J Biol Chem. 2015. PMID: 25752610 Free PMC article.
-
Biosynthesis of the urease metallocenter.
Farrugia MA, Macomber L, Hausinger RP. Farrugia MA, et al. J Biol Chem. 2013 May 10;288(19):13178-85. doi: 10.1074/jbc.R112.446526. Epub 2013 Mar 28. J Biol Chem. 2013. PMID: 23539618 Free PMC article. Review.
-
Moving nickel along the hydrogenase-urease maturation pathway.
Tsang KL, Wong KB. Tsang KL, et al. Metallomics. 2022 May 13;14(5):mfac003. doi: 10.1093/mtomcs/mfac003. Metallomics. 2022. PMID: 35556134 Review.
Cited by
-
Protein interactions in human pathogens revealed through deep learning.
Humphreys IR, Zhang J, Baek M, Wang Y, Krishnakumar A, Pei J, Anishchenko I, Tower CA, Jackson BA, Warrier T, Hung DT, Peterson SB, Mougous JD, Cong Q, Baker D. Humphreys IR, et al. Nat Microbiol. 2024 Oct;9(10):2642-2652. doi: 10.1038/s41564-024-01791-x. Epub 2024 Sep 18. Nat Microbiol. 2024. PMID: 39294458 Free PMC article.
-
Li C, Huang P, Wong K, Xu Y, Tan L, Chen H, Lu Q, Luo C, Tam C, Zhu L, Su Z, Xie J. Li C, et al. J Enzyme Inhib Med Chem. 2018 Dec;33(1):1362-1375. doi: 10.1080/14756366.2018.1501044. J Enzyme Inhib Med Chem. 2018. PMID: 30191728 Free PMC article.
-
Eschweiler JD, Farrugia MA, Dixit SM, Hausinger RP, Ruotolo BT. Eschweiler JD, et al. Structure. 2018 Apr 3;26(4):599-606.e3. doi: 10.1016/j.str.2018.03.001. Epub 2018 Mar 22. Structure. 2018. PMID: 29576318 Free PMC article.
-
Zambelli B, Banaszak K, Merloni A, Kiliszek A, Rypniewski W, Ciurli S. Zambelli B, et al. J Biol Inorg Chem. 2013 Dec;18(8):1005-17. doi: 10.1007/s00775-013-1049-6. Epub 2013 Oct 15. J Biol Inorg Chem. 2013. PMID: 24126709
-
Jaburetox: update on a urease-derived peptide.
Becker-Ritt AB, Portugal CS, Carlini CR. Becker-Ritt AB, et al. J Venom Anim Toxins Incl Trop Dis. 2017 Jun 15;23:32. doi: 10.1186/s40409-017-0122-y. eCollection 2017. J Venom Anim Toxins Incl Trop Dis. 2017. PMID: 28638403 Free PMC article. Review.
References
Publication types
MeSH terms
Substances
LinkOut - more resources
Full Text Sources