Reevaluating carbon fluxes in subduction zones, what goes down, mostly comes up - PubMed
- ️Thu Jan 01 2015
Reevaluating carbon fluxes in subduction zones, what goes down, mostly comes up
Peter B Kelemen et al. Proc Natl Acad Sci U S A. 2015.
Abstract
Carbon fluxes in subduction zones can be better constrained by including new estimates of carbon concentration in subducting mantle peridotites, consideration of carbonate solubility in aqueous fluid along subduction geotherms, and diapirism of carbon-bearing metasediments. Whereas previous studies concluded that about half the subducting carbon is returned to the convecting mantle, we find that relatively little carbon may be recycled. If so, input from subduction zones into the overlying plate is larger than output from arc volcanoes plus diffuse venting, and substantial quantities of carbon are stored in the mantle lithosphere and crust. Also, if the subduction zone carbon cycle is nearly closed on time scales of 5-10 Ma, then the carbon content of the mantle lithosphere + crust + ocean + atmosphere must be increasing. Such an increase is consistent with inferences from noble gas data. Carbon in diamonds, which may have been recycled into the convecting mantle, is a small fraction of the global carbon inventory.
Keywords: aqueous geochemistry; carbon cycle; metasediment diapirs; peridotite carbonation; subduction.
Conflict of interest statement
The authors declare no conflict of interest.
Figures
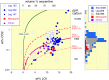
Relationship of loss-on-ignition (LOI) to [CO2] (left axis) and [C] (right axis) in altered mantle peridotites from ophiolites and midocean ridges. Top scale translates LOI to volume percent serpentine, assuming LOI is mainly H2O in serpentine with a density of 2,485 kg/m3, and prealteration density is 3,300 kg/m3. Contours show linear relationships between LOI and [CO2], with intercept = 0 and slopes given on the left axis in red. Data sources in Dataset S3.
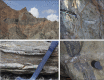
Examples of carbon-bearing rocks formed in subduction zones. (Top Left) Listvenites about 500 m above the basal thrust of the Samail ophiolite in Oman (36). (Top Right) Talc–carbonate rock near Little Harbor, Santa Catalina, CA. (Bottom Left) Marble in eclogite facies metasediments underlying the Higashi-Akaishi mantle peridotite, Japan. (Bottom Right) Magnesite-bearing dunite vein cutting chromitite band in in the Higashi-Akaishi peridotite.
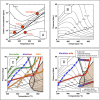
Solubility of CaCO3 in aqueous fluids (see SI Text for calculation methods and references). (A) Isobars of CaCO3 solubility as a function of T. Red circles indicate PT solubility conditions for Santa Catalina; the basal thrust of the Trinity peridotite; lawsonite blueschists; and eclogite to UHP metamorphic terrains such as western Norway and the Higashi-Akaishi peridotite, Japan. (B) Contours of [C] in parts per million for aqueous fluids saturated in CaCO3 as a function of P and T. (C) B overlain by 25× subduction zone geotherms of Syracuse et al. (39). Dashed lines, base of subducting oceanic crust; solid lines, top of subducting volcanics and sediments; green, Kermadec; blue and red, Aleutians; orange, southern Chile. Solid gray curve indicates stability limit of serpentine (118). Orange field indicates PT conditions above the fluid-saturated solidus for metabasalt and metasediment (69). (D) B with Aleutian geotherms. Upper arrow, PT trajectory for fluids produced by serpentine dehydration; lower arrow, PT trajectory for fluids rising into the mantle lithosphere and crust.
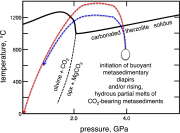
PT trajectory for partial melts of subducting material and for buoyant diapirs. Melting of metabasalt and most metasediments, and melting along peridotite–marble contacts, will produce CO2-bearing silicate melt. Reaction of these melts with peridotite will produce a range of CO2+H2O-bearing liquids from andesite to alkali basalt to carbonatite. CO2-rich, H2O-poor melts will crystallize and evolve CO2-rich fluid at the carbonated lherzolite solidus (refs. and and additional references in SI Text). Hydrous melts will exsolve CO2-rich fluids. Remaining melts and fluids will ascend past the carbonated lherzolite solidus and form carbon-bearing minerals in the mantle lithosphere and crust.
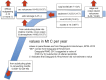
Major fluxes of carbon estimated in this paper, with values from Dasgupta and Hirschmann (1) for comparison.
Similar articles
-
Slab melting as a barrier to deep carbon subduction.
Thomson AR, Walter MJ, Kohn SC, Brooker RA. Thomson AR, et al. Nature. 2016 Jan 7;529(7584):76-9. doi: 10.1038/nature16174. Nature. 2016. PMID: 26738593
-
Tracking carbon from subduction to outgassing along the Aleutian-Alaska Volcanic Arc.
Lopez T, Fischer TP, Plank T, Malinverno A, Rizzo AL, Rasmussen DJ, Cottrell E, Werner C, Kern C, Bergfeld D, Ilanko T, Andrys JL, Kelley KA. Lopez T, et al. Sci Adv. 2023 Jun 28;9(26):eadf3024. doi: 10.1126/sciadv.adf3024. Epub 2023 Jun 28. Sci Adv. 2023. PMID: 37379389
-
Carbonate-rich crust subduction drives the deep carbon and chlorine cycles.
Chen C, Förster MW, Foley SF, Shcheka SS. Chen C, et al. Nature. 2023 Aug;620(7974):576-581. doi: 10.1038/s41586-023-06211-4. Epub 2023 Aug 9. Nature. 2023. PMID: 37558874
-
Plank T, Manning CE. Plank T, et al. Nature. 2019 Oct;574(7778):343-352. doi: 10.1038/s41586-019-1643-z. Epub 2019 Oct 16. Nature. 2019. PMID: 31619791 Review.
-
Foley SF, Chen C, Jacob DE. Foley SF, et al. Natl Sci Rev. 2024 Mar 18;11(6):nwae098. doi: 10.1093/nsr/nwae098. eCollection 2024 Jun. Natl Sci Rev. 2024. PMID: 38933600 Free PMC article. Review.
Cited by
-
Deep carbon recycling viewed from global plate tectonics.
Zhang M, Xu S, Sano Y. Zhang M, et al. Natl Sci Rev. 2024 Apr 12;11(6):nwae089. doi: 10.1093/nsr/nwae089. eCollection 2024 Jun. Natl Sci Rev. 2024. PMID: 38933601 Free PMC article. Review.
-
Olivine alteration and the loss of Mars' early atmospheric carbon.
Murray J, Jagoutz O. Murray J, et al. Sci Adv. 2024 Sep 27;10(39):eadm8443. doi: 10.1126/sciadv.adm8443. Epub 2024 Sep 25. Sci Adv. 2024. PMID: 39321300 Free PMC article.
-
Redox-freezing and nucleation of diamond via magnetite formation in the Earth's mantle.
Jacob DE, Piazolo S, Schreiber A, Trimby P. Jacob DE, et al. Nat Commun. 2016 Jun 21;7:11891. doi: 10.1038/ncomms11891. Nat Commun. 2016. PMID: 27327434 Free PMC article.
-
Shear heating reconciles thermal models with the metamorphic rock record of subduction.
Kohn MJ, Castro AE, Kerswell BC, Ranero CR, Spear FS. Kohn MJ, et al. Proc Natl Acad Sci U S A. 2018 Nov 13;115(46):11706-11711. doi: 10.1073/pnas.1809962115. Epub 2018 Oct 29. Proc Natl Acad Sci U S A. 2018. PMID: 30373832 Free PMC article.
-
Volatile-consuming reactions fracture rocks and self-accelerate fluid flow in the lithosphere.
Uno M, Koyanagawa K, Kasahara H, Okamoto A, Tsuchiya N. Uno M, et al. Proc Natl Acad Sci U S A. 2022 Jan 18;119(3):e2110776118. doi: 10.1073/pnas.2110776118. Proc Natl Acad Sci U S A. 2022. PMID: 35031568 Free PMC article.
References
-
- Dasgupta R, Hirschmann MM. The deep carbon cycle and melting in Earth's interior. Earth Planet Sci Lett. 2010;298:1–13.
-
- Dasgupta R. Ingassing, storage, and outgassing of terrestrial carbon through geologic time. Rev Mineral Geochem. 2013;75:183–229.
-
- Alt JC, et al. The role of serpentinites in cycling of carbon and sulfur: Seafloor serpentinization and subduction metamorphism. Lithos. 2013;178:40–54.
-
- Jarrard RD. Subduction fluxes of water, carbon dioxide, chlorine, and potassium. Geochem Geophys Geosyst. 2004;4(5):8905.
-
- Burton MR, Sawyer GM, Granieri D. Deep carbon emissions from volcanoes. Rev Mineral Geochem. 2013;75:323–354.
Publication types
LinkOut - more resources
Full Text Sources
Other Literature Sources