The Chloroplast Land Plant Phylogeny: Analyses Employing Better-Fitting Tree- and Site-Heterogeneous Composition Models - PubMed
- ️Wed Jan 01 2020
The Chloroplast Land Plant Phylogeny: Analyses Employing Better-Fitting Tree- and Site-Heterogeneous Composition Models
Filipe Sousa et al. Front Plant Sci. 2020.
Abstract
The colonization of land by descendants of charophyte green algae marked a turning point in Earth history that enabled the development of the diverse terrestrial ecosystems we see today. Early land plants diversified into three gametophyte-dominant lineages, namely the hornworts, liverworts, and mosses, collectively known as bryophytes, and a sporophyte-dominant lineage, the vascular plants, or tracheophytes. In recent decades, the prevailing view of evolutionary relationships among these four lineages has been that the tracheophytes were derived from a bryophyte ancestor. However, recent phylogenetic evidence has suggested that bryophytes are monophyletic, and thus that the first split among land plants gave rise to the lineages that today we recognize as the bryophytes and tracheophytes. We present a phylogenetic analysis of chloroplast protein-coding data that also supports the monophyly of bryophytes. This newly compiled data set consists of 83 chloroplast genes sampled across 30 taxa that include chlorophytes and charophytes, including four members of the Zygnematophyceae, and land plants, that were sampled following a balanced representation of the main bryophyte and tracheophyte lineages. Analyses of non-synonymous site nucleotide data and amino acid translation data result in congruent phylogenetic trees showing the monophyly of bryophytes, with the Zygnematophyceae as the charophyte group most closely related to land plants. Analyses showing that bryophytes and tracheophytes evolved separately from a common terrestrial ancestor have profound implications for the way we understand the evolution of plant life cycles on land and how we interpret the early land plant fossil record.
Keywords: bryophytes; chloroplast; composition heterogeneity; land plants; phylogeny.
Copyright © 2020 Sousa, Civáň, Foster and Cox.
Figures
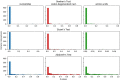
Plots of p-values for Bowker's, Stuart's, and Ababneh's matched-pairs tests of model homogeneity for each of the three data sets. Numbers of rejected (p-values < 0.05) tests: Bowker's test nucleotides 427 (98%), codon-degenerated nucl. 398 (91%), amino acids 401 (92%); Stuart's test nucleotides 424 (97%), codon-degenerated nucl. 387 (89%), amino acids 401 (98%); Ababneh's test nucleotides 331 (76%), codon-degenerated nucl. 193 (44%), amino acids 126 (29%).
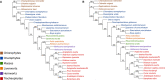
(A) ML bootstrap analysis (400 replicates) of the nucleotide data (GTR+Γ4). Optimal ML tree score: -ln L= 757308.3933. Length of optimal tree = 10.0 substitutions/site. (B) ML bootstrap analyses (400 replicates) of the codon-degenerate recoded nucleotide data (GTR+Γ4). Optimal ML tree score: -ln L= 304862.2078. Optimal tree length = 2.7 substitutions/site.
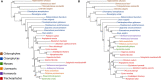
Bayesian MCMC tree-heterogeneous composition analyses of the amino acid data (gcpREV+Γ4+FNDCH2) (A) Run1: Marginal likelihood: Lh= 302615.5702. Posterior predictive simulations of χ2 statistic of composition homogeneity: original statistic = 3021.4465, sample distribution = 2040.7165 to 3069.3241, p-value= 0.0005. 4,000,000 generations, 40,000 samples, 10,000 discarded as burnin. Mean tree length = 5.6538 substitutions/site. (B) Run2: Marginal likelihood: Lh= 302634.1013. Posterior predictive simulations of χ2 statistic of composition homogeneity: original statistic= 3021.4465, sample distribution = 2047.7021 to 3081.9710, p-value = 0.0002. 4,000,000 generations, 40,000 samples, 10,000 discarded as burnin. Mean tree length = 5.6872 substitutions/site.
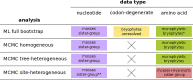
A summary of bryophyte relationships obtained from nucleotide, codon-degenerate, and amino acid translation data using Maximum-likelihood bootstrap analyses (ML) and Bayesian (MCMC) homogeneous, tree-heterogeneous, and site-heterogeneous analyses. Tree nodes were considered supported if the bootstrap value was equal or higher than 80% or if the posterior probability was equal or higher than 95%. (*) part of the analyses without node support; (**) no node support.
Similar articles
-
Liu Y, Cox CJ, Wang W, Goffinet B. Liu Y, et al. Syst Biol. 2014 Nov;63(6):862-78. doi: 10.1093/sysbio/syu049. Epub 2014 Jul 28. Syst Biol. 2014. PMID: 25070972
-
Nuclear protein phylogenies support the monophyly of the three bryophyte groups (Bryophyta Schimp.).
de Sousa F, Foster PG, Donoghue PCJ, Schneider H, Cox CJ. de Sousa F, et al. New Phytol. 2019 Apr;222(1):565-575. doi: 10.1111/nph.15587. Epub 2018 Dec 7. New Phytol. 2019. PMID: 30411803
-
TRANSITION TO A LAND FLORA: PHYLOGENETIC RELATIONSHIPS OF THE GREEN ALGAE AND BRYOPHYTES.
Mishler BD, Churchill SP. Mishler BD, et al. Cladistics. 1985 Sep;1(4):305-328. doi: 10.1111/j.1096-0031.1985.tb00431.x. Cladistics. 1985. PMID: 34965680
-
Major transitions in the evolution of early land plants: a bryological perspective.
Ligrone R, Duckett JG, Renzaglia KS. Ligrone R, et al. Ann Bot. 2012 Apr;109(5):851-71. doi: 10.1093/aob/mcs017. Epub 2012 Feb 22. Ann Bot. 2012. PMID: 22356739 Free PMC article. Review.
-
Vegetative and reproductive innovations of early land plants: implications for a unified phylogeny.
Renzaglia KS, Duff RJT, Nickrent DL, Garbary DJ. Renzaglia KS, et al. Philos Trans R Soc Lond B Biol Sci. 2000 Jun 29;355(1398):769-93. doi: 10.1098/rstb.2000.0615. Philos Trans R Soc Lond B Biol Sci. 2000. PMID: 10905609 Free PMC article. Review.
Cited by
-
Tyszka AS, Bretz EC, Robertson HM, Woodcock-Girard MD, Ramanauskas K, Larson DA, Stull GW, Walker JF. Tyszka AS, et al. Front Plant Sci. 2023 Mar 30;14:1125107. doi: 10.3389/fpls.2023.1125107. eCollection 2023. Front Plant Sci. 2023. PMID: 37063179 Free PMC article.
-
Kato M, Yamamori L, Imada Y, Sota T. Kato M, et al. Proc Biol Sci. 2023 Jun 14;290(2000):20222347. doi: 10.1098/rspb.2022.2347. Epub 2023 Jun 7. Proc Biol Sci. 2023. PMID: 37282533 Free PMC article.
-
Sadamitsu A, Inoue Y, Sakakibara K, Tsubota H, Yamaguchi T, Deguchi H, Nishiyama T, Shimamura M. Sadamitsu A, et al. Plant Mol Biol. 2021 Nov;107(4-5):431-449. doi: 10.1007/s11103-021-01214-z. Epub 2021 Nov 24. Plant Mol Biol. 2021. PMID: 34817767
-
Harris BJ, Clark JW, Schrempf D, Szöllősi GJ, Donoghue PCJ, Hetherington AM, Williams TA. Harris BJ, et al. Nat Ecol Evol. 2022 Nov;6(11):1634-1643. doi: 10.1038/s41559-022-01885-x. Epub 2022 Sep 29. Nat Ecol Evol. 2022. PMID: 36175544 Free PMC article.
-
Lost in the bloom: DNA-PKcs in green plants.
Kumar KRR. Kumar KRR. Front Plant Sci. 2023 Jul 28;14:1231678. doi: 10.3389/fpls.2023.1231678. eCollection 2023. Front Plant Sci. 2023. PMID: 37575944 Free PMC article.
References
LinkOut - more resources
Full Text Sources