Structural basis for selective inhibition of human serine hydroxymethyltransferase by secondary bile acid conjugate - PubMed
- ️Fri Jan 01 2021
Structural basis for selective inhibition of human serine hydroxymethyltransferase by secondary bile acid conjugate
Tomoki Ota et al. iScience. 2021.
Abstract
Bile acids are metabolites of cholesterol that facilitate lipid digestion and absorption in the small bowel. Bile acids work as agonists of receptors to regulate their own metabolism. Bile acids also regulate other biological systems such as sugar metabolism, intestinal multidrug resistance, and adaptive immunity. However, numerous physiological roles of bile acids remain undetermined. In this study, we solved the crystal structure of human serine hydroxymethyltransferase (hSHMT) in complex with an endogenous secondary bile acid glycine conjugate. The specific interaction between hSHMT and the ligand was demonstrated using mutational analyses, biophysical measurements, and structure-activity relationship studies, suggesting that secondary bile acid conjugates may act as modulators of SHMT activity.
Keywords: Metabolic Engineering; Molecular Interaction; Structural Biology.
© 2021 The Author(s).
Conflict of interest statement
The authors declare no competing interests.
Figures
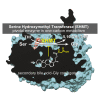
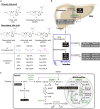
Human bile acid metabolism and one-carbon metabolism (A) Chemical structures of human bile acids. (B) Schematic illustration of bile acid metabolism. CYP = cytochrome P450, BACS = bile acid-CoA synthetase, BAT = bile acid CoA-amino acid N-acyltransferase, BSH = bile salt hydrolase. (C) Schematic illustration of cellular one-carbon metabolism. 3-PG = 3-phosphoglycerate, PHGDH = phosphoglycerate dehydrogenase, PSAT1 = phosphoserine aminotransferase 1, PSPH = phosphoserine phosphatase, SHMT = serine hydroxymethyltransferase, MTHFD = methylenetetrahydrofolate dehydrogenase-cyclohydrolase, CH2-THF = N-5, N-10-methylenetetrahydrofolate, CH+-THF = 5,10-methenyltetrahydrofolate, CHO-THF = 10-formyltetrahydrofolate, NADP+ = nicotinamide adenine dinucleotide phosphate, NADPH = NADP+ reduced form.
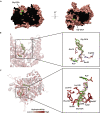
Crystal structure of hSHMT1-Gly-DCA complex (A) Surface representation of the hSHMT1 dimer from two different perspectives. Gly-DCA is displayed as spherical model with carbon shown in green. (B) Interaction between hSHMT1 and Gly-DCA. Gly-DCA is shown as a stick model with carbon in green, whereas hydrogen bonding is represented as red dotted line. 2mFo-DFc electron density of Gly-DCA at the contour level 1.0 σ is shown as a raspberry mesh. Polder map version is shown in Figure S2A. (C) Protein mapping of hSHMT1 using the Eisenberg hydrophobic scale. Polder map version is shown in Figure S2B.
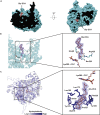
Crystal structure of hSHMT2-Gly-DCA complex (A) Surface representation of the hSHMT2 dimer from two different perspectives. Gly-DCA is shown as a spherical model with carbon displayed in salmon. (B) Interaction between hSHMT2 and Gly-DCA. Gly-DCA is shown as a stick model with carbon displayed in salmon, whereas hydrogen bonding and salt bridge are represented as red dotted line and black dotted line, respectively. 2mFo-DFc electronic density of Gly-DCA at the contour level 1.0 σ is displayed as purple mesh. Polder map version is shown in Figure S3A. (C) Protein mapping of hSHMT2 using the Eisenberg hydrophobic scale. Polder map version is shown in Figure S3B.
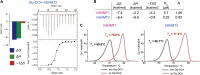
Energetic basis for hSHMT-Gly-DCA interactions (A) The binding of hSHMT2 with Gly-DCA assessed using isothermal titration calorimetry (ITC). ITC was conducted at 37°C in 10 mM HEPES pH 7.5, 200 mM NaCl, 0.5 mM EDTA, 2 mM β-mercaptoethanol, 50 μM PLP, and 3% (v/v) DMSO. (B) ITC profiles of hSHMT1-Gly-DCA and hSHMT2-Gly-DCA interactions. Calorimetric data for hSHMT1 were obtained from our previous report (Nonaka et al., 2019). (C) The binding of Gly-DCA with hSHMT1 or hSHMT2 assessed using differential scanning calorimetry (DSC). DSC was conducted at the speed of 1°C/min in 10 mM HEPES pH 7.5, NaCl (100 mM for hSHMT1 and 200 mM for hSHMT2), 0.5 mM EDTA, 2 mM β-mercaptoethanol, 50 μM PLP (used only for hSHMT2), 18 μM hSHMT1 or hSHMT2 (1.0 mg/mL each), 50 μM Gly-DCA, and 1% (v/v) DMSO. The concentrations of salt and PLP differed slightly for hSHMT1 (100 mM NaCl and 0 μM PLP) and hSHMT2 (200 mM NaCl and 50 μM PLP). This is because hSHMT2 tends to aggregate and release PLP under the conditions used for hSHMT1.
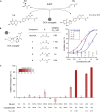
Structure-activity relationship (A) Serine-glycine conversion catalyzed by SHMT. THF = tetrahydrofolate, 5,10-CH2-THF = N-5, N-10-methylenetetrahydrofolate. The carbon atom that is transferred from Ser to THF is shown as red dot. (B) Structure expansion for the bile acid conjugate moiety. Assay conditions: 10 mM HEPES pH 7.5, 100 mM NaCl, 0.5 mM EDTA, 1 mM DTT, 1 mM L-Ser, 0.2 mM THF, 1% (v/v) DMSO. Error bars represent SD, n = 3. (C) Heatmap and bar graph representing the inhibitory effect of human bile acids against hSHMT2. Assay conditions: 10 mM HEPES pH 7.5, 100 mM NaCl, 0.5 mM EDTA, 1 mM DTT, 1 mM L-Ser, 0.2 mM THF, 5 μM compound, 1% (v/v) DMSO. Error bars represent SD, n = 3. IC50 values by Gly/Tau-DCA and Gly/Tau-LCA are shown below the bar graph.
Similar articles
-
Scarsdale JN, Radaev S, Kazanina G, Schirch V, Wright HT. Scarsdale JN, et al. J Mol Biol. 2000 Feb 11;296(1):155-68. doi: 10.1006/jmbi.1999.3453. J Mol Biol. 2000. PMID: 10656824
-
Renwick SB, Snell K, Baumann U. Renwick SB, et al. Structure. 1998 Sep 15;6(9):1105-16. doi: 10.1016/s0969-2126(98)00112-9. Structure. 1998. PMID: 9753690
-
Pinthong C, Maenpuen S, Amornwatcharapong W, Yuthavong Y, Leartsakulpanich U, Chaiyen P. Pinthong C, et al. FEBS J. 2014 Jun;281(11):2570-83. doi: 10.1111/febs.12803. Epub 2014 Apr 28. FEBS J. 2014. PMID: 24698160
-
Signaling from Intestine to the Host: How Bile Acids Regulate Intestinal and Liver Immunity.
Biagioli M, Carino A. Biagioli M, et al. Handb Exp Pharmacol. 2019;256:95-108. doi: 10.1007/164_2019_225. Handb Exp Pharmacol. 2019. PMID: 31119464 Review.
-
Bile Acid Metabolism and Signaling in Cholestasis, Inflammation, and Cancer.
Li T, Apte U. Li T, et al. Adv Pharmacol. 2015;74:263-302. doi: 10.1016/bs.apha.2015.04.003. Epub 2015 May 27. Adv Pharmacol. 2015. PMID: 26233910 Free PMC article. Review.
Cited by
-
Drago VN, Campos C, Hooper M, Collins A, Gerlits O, Weiss KL, Blakeley MP, Phillips RS, Kovalevsky A. Drago VN, et al. Commun Chem. 2023 Aug 3;6(1):162. doi: 10.1038/s42004-023-00964-9. Commun Chem. 2023. PMID: 37532884 Free PMC article.
-
Luo D, Bai Z, Bai H, Liu N, Han J, Ma C, Wu D, Bai L, Li Z. Luo D, et al. J Adv Res. 2024 Aug;62:59-70. doi: 10.1016/j.jare.2023.10.003. Epub 2023 Oct 10. J Adv Res. 2024. PMID: 37820886 Free PMC article.
References
-
- Ananthanarayanan M., Balasubramanian N., Makishima M., Mangelsdorf D.J., Suchy F.J. Human bile salt export pump promoter is transactivated by the farnesoid X receptor/bile acid receptor. J. Biol. Chem. 2001;276:28857–28865. - PubMed
-
- Deutschmann K., Reich M., Klindt C., Dröge C., Spomer L., Häussinger D., Keitel V. Bile acid receptors in the biliary tree: TGR5 in physiology and disease. Biochim. Biophys. Acta Mol. Basis Dis. 2018;1864:1319–1325. - PubMed
LinkOut - more resources
Full Text Sources
Other Literature Sources
Research Materials